Neutrino interaction: exploring coherent neutrino-nucleus scattering with NUCLEUS
Neutrinos are commonly referred to as "ghost" particles that travel through the universe almost without interacting with the matter they cross. To observe these elementary particles in laboratories, usually enormous detectors with tons of target material are needed. However, for neutrino energies below a few tens of MeV, the observation of a long postulated neutrino interaction at a drastically reduced mass of the target material is possible: The coherent elastic neutrino-nucleus scattering, also called CEvNS.
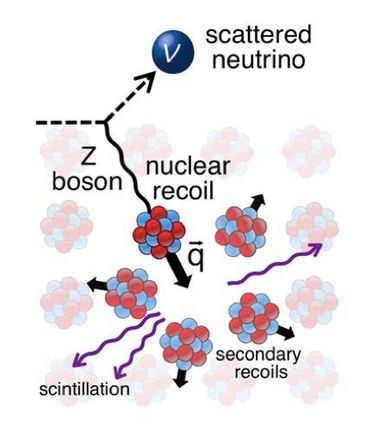
When a neutrino hits a nucleus, the interaction typically depends on the complicated interplay between the neutrino and the individual nucleons. However, if the momentum transfer between the neutrino and the nucleus is small (the de Broglie wavelength is larger than the size of the nucleus), the neutrino does not 'see' the internal structure of the nucleus, but scatters off the nucleus as a whole. This quantum mechanical effect, which for the first time was theoretically described as a Standard model process already in the early 1970s, is the coherent elastic neutrino-nucleus scattering. The interaction is characterized by an exchange of a Z boson, and, as a neutral current process, is insensitive to the flavor of the incoming neutrino. Its scattering cross-section scales with the square number of neutrons in the target nucleus and is orders of magnitude larger than that of other neutrino interactions such as charged-current interactions, neutrino-electron interactions or inverse beta decays. For heavy nuclei and sufficiently intense neutrino sources, the required detector mass shrinks to only a few kilogramms.
Despite its large scattering cross-section, CEvNS was not observed until 2017, more than 40 years after its theoretical prediction. The challenge is to detect the signatures of the process: tiny nuclear recoils with energies in the 10s to 100s eV. The strategy of choosing a target material with high nuclear mass enhances the scattering cross-section on the one hand but comes at the expense of a smaller recoil energy and thus by a smaller experimental signature at the other.
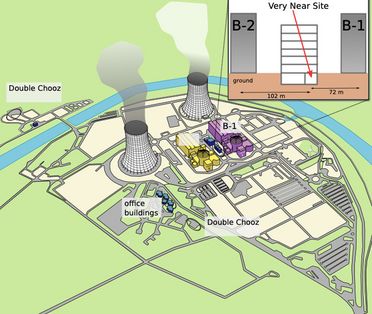
But why are physicists so keen on measuring CEvNS?: First of all, as a Standard model process, CEvNS provides a unique test of the Standard model at low energies itself. Any deviation from the prediction may reveal new physics beyond the established model. Moreover, as CEvNS is insensitive to neutrino flavors, it is a new probe to search for sterile neutrinos. CEvNS offers also a broad spectrum of possible applications in nuclear physics and supernovae detection. As the enhancement of the CEνNS cross-section allows for a miniaturization of neutrino detectors, CEνNS could possibly also be applied for monitoring nuclear reactors.
The NUCLEUS collaboration is currently preparing for a next generation experiment at the Chooz nuclear power plant in France, exploiting the reactor's antineutrino flux to study CEvNS. The experiment will use cryogenic detectors which feature an unprecedentedly low energy threshold to measure the low-energy nuclear recoils of the CEvNS process.
The full exploration of CEvNS requires a detailed understanding of the detector response which currently lacks calibration at lowest energies. Within this SFB, innovative low-energy calibration techniques are developed which will be a milestone of understanding the response of NUCLEUS cryogenic detectors in the region of interest for CEvNS.